Projects
Unlike salamanders, there is currently no regenerative therapy for limb and digit amputation in humans. While prosthetic technologies have dramatically improved, prosthetics fail to restore quality of life to the pre-injury state. The Badylak laboratory has been pursuing regenerative medicine solutions for functional limb and digit regeneration.
Complex tissue regeneration
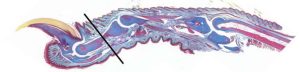
Figure 1: H&E image of mouse digit, with amputation site indicated in black.
The Badylak laboratory has established a non-regenerating mouse model of digit amputation, which is used to study innovative approaches for functional tissue reconstruction, most of which are based upon the inductive effects of extracellular matrix (ECM) (Figure 1) [1,2]. Using this model, we have shown that ECM can positively affect both the recruitment of endogenous stem cells and modulation of the innate immune response.
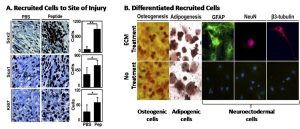
Figure 2: A – In the injured digit, more Sox2+ and Sca1+ cells and more proliferating cells (Ki67+) are present when treated with peptide derived from ECM, as opposed to PBS. B – Isolated cells from the digit tip were shown to be multipotential, differentiating into different cell types in vitro.
Using ECM derived from a porcine urinary bladder matrix (UBM) that was solubilized by pepsin digestion, amputated mouse digits were treated daily for four days and observed the rapid accumulation of a cluster of primitive cells at the site of injury, including multipotential cells with Sox2 and Sca1 markers (Figure 2A). When isolated, these cells were capable of differentiation along osteogenic, adipogenic, and neuronal pathways in vitro (Figure 2B).
The UBM degradation products and solubilized signaling molecules modify the phenotype of macrophages that respond to the digit injury. Using immunofluorescence labeling, a greater ratio of M2, pro-remodeling macrophages to M1, pro-inflammatory macrophages was present at 7 and 14 days after injury. The ability to modulate macrophage phenotype holds significant promise for future tissue remodeling applications. The Badylak laboratory has identified macrophage polarization as a predictor of tissue remodeling outcomes in several clinical applications [3,4].
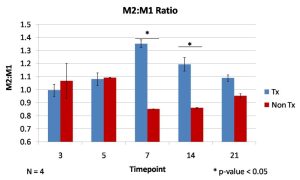
Figure 3: Quantification of immunofluorescence staining for markers of M1 and M2 macrophages was used to characterize the immune response, which has a predominantly more M2:M1 phenotype than the non-treatment control at 7 and 14 days.
While work in the mouse digit model has been promising, questions remain regarding the mechanism by which UBM recruits these progenitor cells to the site of injury. Additionally, methods to promote differentiation of the recruited multipotential cells are being explored.
Presently, a study is in progress to examine the effect of bioactive ECM molecules as well as factors such as pH, O2 concentration, and electrical potential on the functional remodeling of injured soft tissue in a large animal model. The goal of the study is to identify the optimal microenvironment for tissue remodeling and to engineer a delivery device (a Biodome) to monitor and control these factors (Figure 4). Control of the microenvironment through specific compounds delivered via an overlying Biodome will allow for directed differentiation of recruited cells into functional, innervated and vascularized soft tissue.
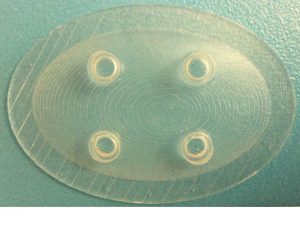
Figure 4: One example of a large animal Biodome prototype.
References:
Agrawal, V., et al., Epimorphic regeneration approach to tissue replacement in adult mammals. Proc Natl Acad Sci U S A, Feb 2010. 107(8): p. 3351-3355.
Agrawal V, Tottey S, Johnson SA, Freund JM, Siu BF, Badylak SF. Recruitment of Progenitor Cells by an Extracellular Matrix Cryptic Peptide in a Mouse Model of Digit Amputation. Tissue Eng Part A. Vol. 17, No. 19-20, October 2011: 2435-2443.
Badylak, S. F., Valentin, J., Ravindra, A., McCabe, G., Stewart-Akers, A. Macrophage Phenotype as a Determinant of Biologic Scaffold Remodeling. Tissue Eng Part A, 2008 Nov; 14(11):1835-1842.
Brown BN, Londono R, Tottey S, Zhang L, Kukla KA, Wolf MT, Daly KA, Reing JE, Badylak SF. Macrophage phenotype as a predictor of constructive remodeling following the implantation of biologically derived surgical mesh materials. Acta Biomater, 2012 Mar;8(3):978-87.
Biological scaffold materials composed of extracellular matrix (ECM) have been used clinically in regenerative medicine for many years. These biomaterials are made from many different animal, tissue and cell sources, raising concerns about the effect of the immune response to materials from such varied origins (Table 1). Commonly used commercial products come from both allogeneic and xenogeneic sources, yet the well characterized immune responses to whole organ allo or xenotransplantation are not seen in response to these biologic scaffold materials. This is does not mean the biological scaffold materials are immunologically inert. Macrophage and neutrophil infiltration of the ECM is noted immediately post engraftment, and is thought to be correlated with the success of remodeling the implanted material. Allogeneic and xenogeneic epitopes, DNA and damage-associated molecular patterns (PAMPs) present within the biomaterial are also thought to contribute to the host response. The immune response to biologic scaffolds rather than being a deleterious outcome, appears to be a necessary part of the remodeling process.
Left image: Extracellular matrix (ECM) device in a rat soft tissue repair model. The surrounding host tissue (cell nuclei stained blue) interacts directly with the ECM material (autofluorescence in red) through the recruitment of anti-inflammatory (M2) macrophages (orange) and the formation of a site-specific remodeling response.
Right image: Neuromuscular junction (green) staining with alpha-bungarotoxin within an area of regenerating muscle 6 months following SIS-ECM implantation. Muscle fibers are stained red, nuceli are blue.
Table 1: Commercially available extracellular matrix (ECM) scaffolds
Product |
Source |
Tissue |
Company |
AlloDerm | human | skin | Lifecell |
AlloPatch | human | dermis | Musculoskeletal Transplant Foundation |
Avaulta®, CollaMend® | porcine | dermis | BARD |
Axis™ dermis | human | dermis | Mentor |
CuffPatch™ | porcine | SIS | Athrotek |
Graft Jacket® | human | skin | Wright Medical Tech |
Oasis® | porcine | SIS | Healthpoint |
OrthADAPT™, DurADAPT™ | equine | pericardium | Pegasus Biologicals |
Permacol™ | porcine | skin | Tissue Science Laboratories |
Restore™ | porcine | SIS | DePuy |
Surgisis®, Durasis®, Stratasis® | porcine | SIS | Cook SIS |
Suspend™ | human | Fascia lata | Mentor |
TissueMend®, Durepair®, Xenform™, SurgiMend™, PriMatrix™ | Fetal bovine | skin | TEI Biosciences |
Veritas®, Dura-Guard®, Vascu-Guard®, Peri-Guard® | bovine | dermis | Synovis Surgical |
Xelma™ | porcine | Teeth enamel | Molnlycke |
The focus of the Badylak laboratory is to develop an in-depth understanding of the host immune response to ECM biological scaffolds, and in particular the role of xenogeneic antigens, macrophages and DAMPs in directing this response. The Gal (Galα1,3-Galβ1-4GlcNAc-R) epitope is a xenogeneic antigen present on cell surfaces in all species apart from humans and old world monkeys (1-3). The gal epitope plays a major role in facilitating hyperacute rejection of xenotransplants (2), but despite its presence in ECM (4), its role in the host response to biological scaffolds is controversial. Determining the effect of this epitope in host response to biological scaffolds is one of the current focuses of the laboratory.
The presence of macrophages in response to engraftment of ECM has been previously noted, but it is only recently that a correlation between macrophage polarization and successful remodeling of ECM has been determined. A beneficial host response, i.e. remodeling of the implanted ECM and migration of native cells into the area, has been associated with a T helper cell type 2 (Th2) response (5, 6) and with alternatively activated or M2 macrophages (7, 8). Conversely, adverse host response, i.e. a persistent proinflammatory response and development of a foreign body reaction, is correlated with classically activated or M1 macrophages (7, 8). The role of xenoantigens within the ECM scaffold that direct this macrophage polarization and the effect that processing of ECM has on this, is a major area of interest for the Badylak laboratory.
DAMPs or damage-associated molecular patterns, are molecules released by cell death. The DAMP classification encompasses many different types of molecules, which normally have several intracellular and external roles. DAMPs may be pro or anti-inflammatory, and their action may be influenced by redox status of the microenvironment. Several well characterized DAMPs are already known to be part of the ECM, and it is proposed that others may also be present within biologic scaffolds. The characterization of DAMPs within biological scaffolds and their effect on the direction of the immune response is another area of focus for the Badylak laboratory.
References
- Spiro, R. G., and V. D. Bhoyroo. 1984. Occurrence of alpha-D-galactosyl residues in the thyroglobulins from several species. Localization in the saccharide chains of the complex carbohydrate units. J Biol Chem 259:9858-9866. PMID: 6086655
- Cooper, D. K., A. H. Good, E. Koren, R. Oriol, A. J. Malcolm, R. M. Ippolito, F. A. Neethling, Y. Ye, E. Romano, and N. Zuhdi. 1993. Identification of alpha-galactosyl and other carbohydrate epitopes that are bound by human anti-pig antibodies: relevance to discordant xenografting in man. Transpl Immunol 1:198-205. PMID: 7521740
- Galili, U., M. R. Clark, S. B. Shohet, J. Buehler, and B. A. Macher. 1987. Evolutionary relationship between the natural anti-Gal antibody and the Gal alpha 1—-3Gal epitope in primates. Proc Natl Acad Sci U S A 84:1369-1373. PMID: 2434954
- McPherson, T. B., H. Liang, R. D. Record, and S. F. Badylak. 2000. Galalpha(1,3)Gal epitope in porcine small intestinal submucosa. Tissue Eng 6:233-239. PMID: 10941218
- Allman, A. J., T. B. McPherson, S. F. Badylak, L. C. Merrill, B. Kallakury, C. Sheehan, R. H. Raeder, and D. W. Metzger. 2001. Xenogeneic extracellular matrix grafts elicit a TH2-restricted immune response. Transplantation 71:1631-1640. PMID: 11435976
- Allman, A. J., T. B. McPherson, L. C. Merrill, S. F. Badylak, and D. W. Metzger. 2002. The Th2-restricted immune response to xenogeneic small intestinal submucosa does not influence systemic protective immunity to viral and bacterial pathogens. Tissue Eng 8:53-62. PMID: 11886654
- Badylak, S. F., and T. W. Gilbert. 2008. Immune response to biologic scaffold materials. Semin Immunol 20:109-116. PMID: 18083531
- Badylak, S. F., J. E. Valentin, A. K. Ravindra, G. P. McCabe, and A. M. Stewart-Akers. 2008. Macrophage phenotype as a determinant of biologic scaffold remodeling. Tissue Eng Part A 14:1835-1842. PMID: 18950271
- Daly KA, Stewart-Akers AM, Hara H, Ezzelarab M, Long C, Cordero K, Johnson SA, Ayares D, Cooper DK, Badylak SF. Effect of the alphaGal epitope on the response to small intestinal submucosa extracellular matrix in a nonhuman primate model. Tissue Eng Part A. 2009 Dec;15(12):3877-88. PMID: 19563260
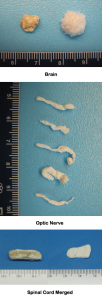
Current research efforts in the Badylak laboratory regarding central nervous system tissue engineering involve:
- The growth of a variety of central nervous system cell types upon biologic scaffolds composed of extracellular matrix (ECM) in vitro,
- The preparation of central nervous system derived extracellular matrix (decellularization of CNS), and
- The application of various forms of extracellular matrix to areas of CNS injury and preclinical animal models.
The objective of our present and future work is to develop an effective substrate for reconstruction of functional central nervous system tissue. We believe that the extracellular matrix provides such a substrate and can “jumpstart” regeneration of central nervous system tissue either by recruitment of endogenous stem cells to the site of injury or by placement of such stem cells within a scaffold that is placed at the injury site.
Peripheral compartment syndrome (PCS) is a major cause of morbidity in soldiers with traumatic extremity injuries. It occurs when the pressure within a muscle compartment exceeds that of the circulation. Acute crush, vascular and orthopedic injuries, which present commonly in military medicine, often lead to the development of PCS. Sequelae of an increase in intra-compartmental pressure include ischemia, necrosis and nerve damage. If untreated, PCS may lead to limb amputation, multiple organ failure and death.
![]() |
|
![]() |
|
Treatment of animal model with ECM results in myogenesis (top), whereas there is only fibrous tissue in fasciotomy treated groups (bottom) after 3 months. |
PCS is characterized by symptoms including pain, pallor, absent pulses, parasthesia and loss of function. It is usually recognised by measurement of the intracompartmental pressure, which is considered diagnostic when it exceeds 30-40 mm Hg. Intracompartmental pressure greater than 12 mm Hg will cause cessation of blood flow in 50% of capillaries (1). Consequently, tissue ischaemia is a significant factor in the pathology of this syndrome. In addition, 6 to 8 hours of tissue ischaemia will result in irreversible damage to the muscle and peripheral nerves (2, 3), which is a serious complicating factor in the long term recovery of function.Peripheral compartment syndrome (PCS) is a major cause of morbidity in soldiers with traumatic extremity injuries. It occurs when the pressure within a muscle compartment exceeds that of the circulation. Acute crush, vascular and orthopedic injuries, which present commonly in military medicine, often lead to the development of PCS. Sequelae of an increase in intra-compartmental pressure include ischemia, necrosis and nerve damage. If untreated, PCS may lead to limb amputation, multiple organ failure and death.
The current best standard of care for PCS is an open fasciotomy, however this procedure is still associated with high levels of morbidity. In addition, a delay of 6-8 hours in treatment increases the incidence of permanent myonecrosis, infection amputation and mortality further (3). Such delays may be common in a military setting (4), making PCS a major concern in soldiers with traumatic extremity injuries. The potential complications and higher level of morbidity associated with the treatment of PCS necessitate the development of better therapeutic options.
A regenerative medicine approach to treatment of PCS may help to decrease the incidence of morbidity and improve the quality of life post injury. The overall goal of such an approach is the reconstitution of functional, vascularized and innervated musculotendinous tissue. Extracellular matrix (ECM) is generated by cells, and provides structural and functional support. Decellularization of cellular material in vitro produces ECM that may be used in vivo to facilitate tissue regeneration. A focus of this project is the development of new treatment modalities for PCS that make use of recent advances in the field of regenerative medicine and the use of biologic scaffolds.
References
- Vollmar, B., S. Westermann, and M. D. Menger. 1999. Microvascular response to compartment syndrome-like external pressure elevation: an in vivo fluorescence microscopic study in the hamster striated muscle. J Trauma 46:91-96. PMID: 9932689
- Goaley, T. J., Jr., A. D. Wyrzykowski, J. B. MacLeod, K. B. Wise, C. J. Dente, J. P. Salomone, J. M. Nicholas, G. A. Vercruysse, W. L. Ingram, G. S. Rozycki, and D. V. Feliciano. 2007. Can secondary extremity compartment syndrome be diagnosed earlier? Am J Surg 194:724-726; discussion 726-727. PMID: 18005761
- Pai, V. 2007. Acute compartment syndrome after rupture of the medial head of gastrocnemius in a child. J Foot Ankle Surg 46:288-290. PMID: 17586443
- Ritenour, A. E., W. C. Dorlac, R. Fang, T. Woods, D. H. Jenkins, S. F. Flaherty, C. E. Wade, and J. B. Holcomb. 2008. Complications after fasciotomy revision and delayed compartment release in combat patients. J Trauma 64:S153-161; discussion S161-152. PMID: 18376159
Esophageal adenocarcinoma is increasing at a faster rate than any other cancer in the United States. Treatment options include a variety of techniques to resect the affected tissue but these strategies have had limited success and invariably have high complication and mortality rates.
To investigate the use of biologic scaffold materials as a regenerative medicine approach to induce esophageal repair after partial or total esophagectomy and/or mucosectomy. |
Extracellular matrix (ECM)-based biomaterials have shown promise for esophageal reconstruction. In early pre-clinical studies ECM scaffolds were used to repair partial and full thickness esophageal defects encompassing various portions of the total esophageal circumference. This initial study showed well organized, fully re-epithelialized site-specific tissue that was contiguous with the native esophagus. However, full circumferential defects healed with stricture within 19 days after surgery [1].
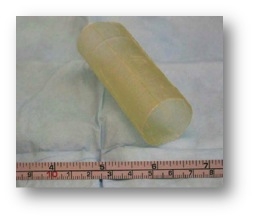
Figure 1: Tubular ECM scaffold used to repair esophageal defects [2]
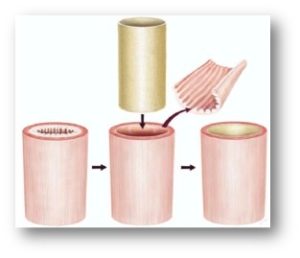
Figure 2: Depiction of surgical procedure used to treat human patients with diseased esophageal muscosa. The mucosal lining is removed and replaced with a tubular ECM scaffold, which leads to restoration of normal tissue.
To facilitate rapid clinical translation of an ECM scaffold approach to esophagus reconstruction, a study was designed to specifically evaluate the efficacy of an ECM scaffold in a surgical procedure that is currently performed, and accepted, namely the “gastric pull-up” procedure [5]. In an animal model, transections were made in the cervical esophagus and at the gastroesophageal junction and a few centimeters of mucosa were also resected. The ECM was placed at the site of the endomucosal resection to reinforce the anastomosis. The shape of the device was customized to the shape of the anatomy (i.e., tube for cervical, funnel shape for GE junction) in each location to reduce the anastomotic tension and disruption of blood supply. The remodeling of the ECM reinforced anastomoses was compared to an experimental control in which the endomucosal resection was performed at the time of anastomosis, and a clinical control in which the mucosa was left intact. After two months, the presence of UBM-ECM resulted in less stenosis and less contracture of the cervical and distal esophagus compared to the control. Furthermore, the site of ECM remodeling showed restoration of a more mature epithelium and regeneration of islands of muscle that bridged the gap between the native muscle tissues on either side of the surgical transection. This study suggests that the use of an ECM scaffold during the gastric pull-up surgery may substantially decrease the rate of complications (i.e., stricture, leaks) associated with this procedure.
![]() |
Figure 3: Diagnostic biopsy (top row), postoperative biopsy (second row). The diagnostic biopsies all show adenocarcinoma. The postoperative biopsies show replacement of the ECM scaffold with mature, differentiated squamous epithelium. Scale bars represent 100 mm. |
Naturally, in order to develop better regenerative medicine therapies for esophageal reconstruction, a more thorough understanding of esophageal disease progression and the mechanisms by which bioscaffolds mitigate the default tissue response to injury is required. The Badylak laboratory is currently working on these two separate but related questions: Specifically, we aim to determine the mechanism by which normal, inflammatory, and cancerous ECM dynamically, and reciprocally, instructs the behavior of esophageal cells and supporting cells (e.g. immune cells) through physical and biochemical cues. The Badylak laboratory is uniquely equipped to conduct this study for its pioneering work in decellularization of mammalian tissues and development of organ-specific hydrogels [8,9]. We are currently working to develop the first hydrogel from inflammatory and cancerous ECM. Furthermore, we aim to temporally resolve the microenvironment-specific tissue-remodeling response sequence after biologic scaffold placement in a rat model of esophageal adenocarcinoma. The findings of this work will improve regenerative medicine strategies for patients with Barrett’s and mucosal adenocarcinoma, and identify biomarkers for clinical surveillance in this increasingly devastating form of cancer.
References:
- Badylak S, Meurling S, Chen M, Spievack A, Simmons-Byrd A. Resorbable bioscaffold for esophageal repair in a dog model. J Pediatr Surg. 2000 Jul;35(7):1097-1103. PMID: 10917304
- Badylak SF, Vorp DA, Spievack AR, Simmons-Byrd A, Hanke J, Freytes DO, Thapa A, Gilbert TW, Nieponice A. Esophageal reconstruction with ECM and muscle tissue in a dog model. J Surg Res. 2005 Sep;128(1):87-97. PMID: 15922361
- Brown B, Lindberg K, Reing J, Stolz DB, Badylak SF. The basement membrane component of biologic scaffolds derived from extracellular matrix. Tissue Eng. 2006 Mar;12(3):519-526. PMID: 16579685
- Nieponice A, Gilbert TW, Badylak SF. Reinforcement of esophageal anastomoses with an extracellular matrix scaffold in a canine model. Ann Thorac Surg. 2006 Dec;82(6):2050-2058. PMID: 17126109
- Nieponice A, McGrath K, Qureshi I, Beckman EJ, Luketich JD, Gilbert TW, Badylak SF. An extracellular matrix scaffold for esophageal stricture prevention after circumferential EMR. Gastrointest Endosc. 2008;doi:10.1016/j.gie.2008.04.022. PMID: 18657808
- Agrawal V, Brown BN, Beattie AJ, Gilbert TW, Badylak SF. Evidence of innervation following extracellular matrix scaffold mediated tissue remodeling. J Tissue Eng Regen Med. 2009 Dec;3(8):590-600. PMID: 19701935
- Badylak SF, Hoppo T, Nieponice A, Gilbert TW, Davison JM, Jobe BA. Esophageal preservation in five male patients after endoscopic inner-layer circumferential resection in the setting of superficial cancer: a regenerative medicine approach with a biologic scaffold. Tissue Eng Part A. 2011;17(11-12): p. 1643- 50. PMID: 21306292
- Wolf MT, Daly KA, Brennan-Pierce EP, Johnson SA, Carruthers CA, D’Amore A, Nagarkar SP, Velankar SS, Badylak SF. A hydrogel derived from decellularized dermal extracellular matrix. Biomaterials. 2012 Oct;33(29):7028-38. doi: 10.1016/j.biomaterials.2012.06.051. Epub 2012 Jul 11. PMID: 22789723
- Medberry CJ, Crapo PM, Siu BF, Carruthers CA, Wolf MT, Nagarkar SP, Agrawal V, Jones KE, Kelly J, Johnson SA, Velankar SS, Watkins SC, Modo M, Badylak SF. Hydrogels derived from central nervous system extracellular matrix. Biomaterials. 2013 Jan;34(4):1033-40. doi: 10.1016/j.biomaterials.2012.10.062. Epub 2012 Nov 16. PMID: 23158935
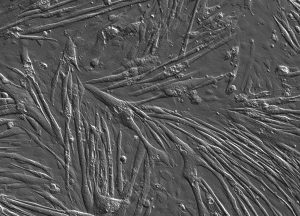
Differentiated C2c12 cells form multinucleated skeletal muscle myotubes in vitro
The reconstruction of skeletal muscle tissue either lost by traumatic injury, tumor ablation or due to congenital abnormalities is hampered by the lack of availability of functional substitutes to this native tissue. Satisfactory repair of a severed muscle relies upon good apposition of the cut ends with regeneration of the motor nerve branches serving the muscle. However, in the case of multiple lacerations or debridement of traumatized muscle there is frequently a loss of muscle tissue. This a particular problem in the context of military combat wounds where the morbidity associated with severe soft tissue injury can be significant, accounting for over 80% of all surgical amputations.
While Skeletal, smooth and cardiac muscle neogenesis has been noted using the ECM bioscaffolds developed in the lab, the source of cells that contribute to this new muscle tissue formation has not been investigated, nor has the full potential of muscle reconstruction been investigated. This project aims to develop an ECM scaffold that can facilitate restoration of a functional skeletal muscle-tendon unit and ultimately a complete muscle compartment.
Our initial studies have focused on the use of small intestinal submucosa scaffolds to replace partial lost gastrocnemius muscle and achillies tendon. These studies have shown that this material is capable of stimulating restoration of significant muscle mass and restitution of the musclulotendinous junction restoring functionality to a damaged limb. This new muscle growth is both contractile and innervated and comprises a mixed muscle fiber population similar to the native muscle that was lost.
Future studies will further develop this implant to replace larger muscular defects. We are identifying appropriate techniques to seed the scaffold with mesechymal stem cells that will allow regeneration of entire muscle units where no native skeletal muscle remains.
The Badylak laboratory contains a full range of equipment for testing both the mechanical characteristics of regenerating muscle including ex vivo tissue baths as well as equipment to determine innervation of the muscle via electromyography both in vivo and ex vivo.
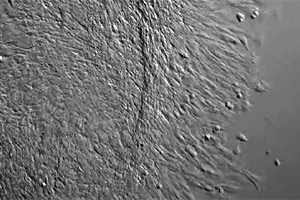
C2C12 myoblasts migrating into a scratch wound in vitro over and 18 hour perido in response to pepsin digested SIS-ECM
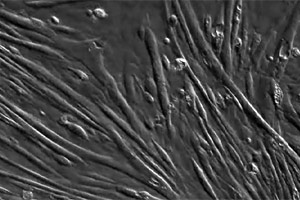
Differentiated C2C12 cells form myotubes in vitro
Extracellular matrix (ECM) represents the structural and functional molecules secreted by the resident cells of every tissue in the body. The Badylak laboratory has extensive experience isolating and harvesting this ECM and producing usable formats for use in pre-clinical animal and bench top studies. We routinely produce ECM from tissue such as small intestine, urinary bladder and liver tissue, typically from porcine sources. The cells of these tissues are removed by physical, enzymatic, and/or chemical methods so that only the native ECM remains. This ECM can then be used as a wet hydrated form, multilaminate sheets, and hydrogel forms.
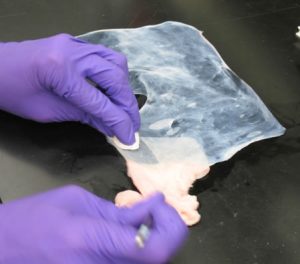
MANUAL removal of muscle layers from porcine urinary bladder
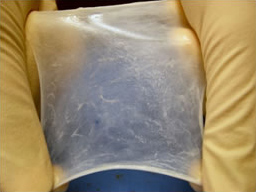
Hydrated ECM
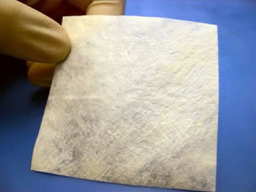
Lyophilized ECM
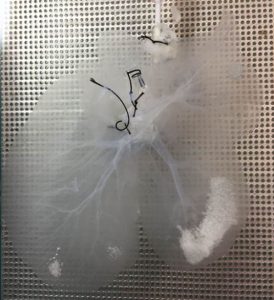
Decellularized porcine liver
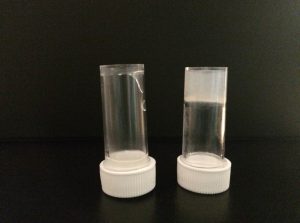
Hydrogel ECM
Organ engineering, as opposed to tissue engineering, poses significant challenges including the requirement for an immediately functional vascular network, functional parenchymal cells, and lymphatic and innervation potential. In recent years a promising approach for functional organ replacement has emerged: the decellularization of whole organs, providing an acellular three-dimensional scaffold composed of extracellular matrix (ECM). Importantly, the scaffold has been shown to retain the native vascular network of the organ (Figure 1).
Recent studies have shown that tissue/organ specific ECMs can support site appropriate cell phenotype and lineage-directed differentiation. It is intuitive that the native 3-dimensional architecture and microenviromental niche provided by the extracellular matrix are important for cell attachment and differentiation. By decellularizing the entire organ, most of the 3D structure of the ECM can be retained to provide a native framework for organ reconstruction.
The long term goal of this work is to establish the decellularization, recellularization with autologous cells (thus avoiding the need for subsequent immunosuppression), and transplantation criteria necessary to produce functional bioengineered organs for clinical translation.
The Badylak lab specifically focuses on whole liver and heart regeneration.
Engineering a Liver
To engineer a functional whole organ liver for treatment of end stage liver disease.
Approximately 27,000 deaths occur annually in the United States alone from end-stage liver disease. We are aggressively investigating a therapeutic whole organ replacement strategy that is based upon the concept that functional hepatic tissue can be engineered by the effective delivery of autologous hepatic parenchymal and non-parenchymal cells within a xenogeneic 3-dimensional scaffold composed of liver extracellular matrix (3D L-ECM). This approach would include immediate in-situ transplantation of the cell seeded scaffold, providing the requisite perfusion by the host circulation that supplies appropriate microenvironmental cues, nutrients, and signaling factors necessary for liver regeneration.
![]() |
Figure 1: Snapshots of blue dye flowing through the vasculature of a three-dimensional rat liver ECM scaffold. |
Recent studies have shown that tissue/organ specific ECMs can support site appropriate cell phenotype and lineage-directed differentiation. It is intuitive that the native 3-dimensional architecture and microenviromental niche provided by the extracellular matrix are important for cell attachment and differentiation.
By decellularizing the entire organ, most of the 3D structure of the ECM can be retained to provide a native framework for organ reconstruction. The long term goal of this work is to establish the decellularization, recellularization and transplantation criteria necessary to produce a fully functional bioengineered liver for organ transplantation and drug discovery.
Engineering a Heart
The use of Cardiac ECM (C-ECM) versus ECM derived for other tissue sources may promote faster myocardial reconstruction of functional tissue due to similar structure and function.
Our laboratory has shown that an entire porcine heart can be decellularized thus providing a platform for whole organ engineering (Fig. 2) [1]. The resultant decellularized heart provides a three-dimensional scaffold and microenvironment to support site-appropriate cell differentiation and spatial organization. The decellularization protocol is completed in less than 10 hours using pulsatile retrograde aortic perfusion with serial perfusion of an enzymatic, nonionic detergent, ionic detergent, and acid solution with hypotonic and hypertonic rinses to systemically remove cellular content. The resultant c-ECM retains collagen, elastin, glycosaminoglycans, and mechanical integrity.
![]() |
Figure 2: Representative images of the gross appearance of intact porcine hearts subjected to decellularization by retrograde perfusion. |
Our laboratory subsequently evaluated a patch of this c-ECM in a full-thickness right ventricle outflow tract repair compared to an inert Dacron in a rat model up to 4 months [2]. The Dacron patch was encapsulated with a fibrous capsule with minimal cellular infiltration, while the c-ECM patch remodeled into a dense connective tissue with scattered islands of striated cardiomyocytes (Fig. 3) and showed superior echocardiography results. This response was consistent with a previous study by our laboratory in which a decellularized biological scaffold derived from porcine bladder tissue was compared to a synthetic material for an LV-free wall infarction in a porcine animal model up to 3 months [3]. However, the constructive remodeling responses are not fully understood and are the subject of present study.
![]() |
Figure 3: Immunofluorescent staining of a Dacron and cECM right ventricle explant. The Dacron patch explant had an intact endothelium but no cellular infiltration while the cECM patch explant had cellular infiltration with striation. |
While these results are promising, the mechanism by which ECM promotes a constructive remodeling response is only partially understood. Further, the term ECM is not used in a consistent manner in the literature – there are different tissue sources and protocols that may result in different structures and fractions of remnant constituent proteins. Our laboratory is currently performing an extensive in-vitro characterization of the structure and cell-ECM interaction on ECM derived from cardiac and other tissue types treated with different detergents. Both cardiac and non-cardiac derived stem cells will be used to assess if the ‘seed’ or ‘soil’ is critical to a constructive remodeling response in cardiac tissue. The findings will be used to define the optimal tissue type and detergent treatment for a cardiac site-specific constructive remodeling response.
References:
- Robinson KA, Li J, Mathison M, Redkar A, Cui J, Chronos NA, Matheny RG, Badylak SF. Extracellular Matrix Scaffold for Cardiac Repair. Circulation. 2005 Aug 30;112(9 Suppl):I135-43. PMID: 16256789
- Wainwright JM, Czajka CA, Patel UB, Freytes DO, Tobita K, Gilbert TW, Badylak SF. Preparation of Cardiac Extracellular Matrix from an Intact Porcine Heart. Tissue Engineering Part C: Methods. 2009;16(3):525-532. PMID: 19702513
- Wainwright JM, Hashizume R, Fujimoto KL, Remlinger NT, Pesyna C, Wagner WR, Tobita K, Gilbert TW, Badylak SF. Right Ventricular Outflow Tract Repair with a Cardiac Biologic Scaffold. Cells Tissues Organs. 2011;15219:1-12. PMID: 22025093