October 2020 | VOL. 19, NO. 10| www.McGowan.pitt.edu
Dr. Alejandro Soto-Gutierrez Receives NIH Funding to Use Tiny, Bioengineered Organ Models to Improve Clinical Trials’ Development and Design
Approximately 85% of late-stage clinical trials of candidate drugs fail because of drug safety problems or ineffectiveness, despite promising preclinical test results. To help improve the design and implementation of clinical trials, the National Institutes of Health has awarded 10 grants to support researchers’ efforts in using tiny, bioengineered models of human tissues and organ systems to study diseases and test drugs. One major goal of the funded projects is to develop ways to better predict which patients are most likely to benefit from an investigational therapy prior to initiating clinical trials. McGowan Institute for Regenerative Medicine affiliated faculty member Alejandro Soto-Gutierrez, MD, PhD, Associate Professor in the Department of Pathology at the University of Pittsburgh and an affiliated faculty member of the Children’s Hospital of Pittsburgh of UPMC and the Thomas E. Starzl Transplantation Institute, is a co-principal investigator on one of the 10 recently funded projects.
The team’s project is entitled, “A Vascularized Patient-Derived iPSC Liver Acinus Microphysiological System as an Innovative Precision Medicine Platform for Optimizing Clinical Trial Design for Nonalcoholic Fatty Liver Disease.” Non-alcoholic fatty liver disease (NAFLD) is a major health crisis with no approved therapeutics and many failures in the clinic. A vascularized patient-derived liver acinus microphysiology system (vLAMPS) is an innovative precision medicine platform for optimizing clinical trial design for NAFLD. The research team will ultimately generate patient-specific iPSCs of the four cell types from patients in a NAFLD clinic to create patient-specific vLAMPS. The researchers will test two cohorts: 1) patients with the PNPLA3 I 148M variant and 2) patients with the wild-type PNPLA3, to identify the patients who respond to two NAFLD drugs that have or are now going through clinical trials and two control drugs. Importantly, this paradigm circumvents the conundrum of high-risk patients being enrolled in large prolonged studies with a high likelihood of failure being simultaneously disqualified from other potentially beneficial studies/treatments. This approach will prove transformational for clinical trial design by enriching for subjects most likely to benefit from a therapy, and in the future, after more than one currently investigational drugs are approved, for precision medicine to identify the most efficacious therapy for high-risk subgroups.
The project funding is $748,638 and the project period is July 15, 2020-June 30, 2021.
RESOURCES AT THE MCGOWAN INSTITUTE
November Histology Special
The Herovici combination of aniline blue or picro methyl blue and picro acid fuchsin results in Type I mature collagen staining red while reticulum and newly formed Type III collagen stains blue.
You’ll receive 25% off your Herovici staining in November when you mention this ad. Contact Julia at the McGowan Core Histology Lab by email: Hartj5@upmc.edu or call 412-624-5265.
New Sample Submission Procedures: In response to COVID-19, we ask that you contact us to schedule a drop off time. When you arrive at the building you can call our laboratory at (412)624-5365. Someone will meet you in the lobby to collect your samples. When your samples are completed, you will receive an email to schedule a pickup time.
SCIENTIFIC ADVANCES
Monitoring Coronary Artery Disease in Real-Time
Coronary artery disease – a leading cause of death in the US – narrows or blocks arteries that carry a vital supply of blood, oxygen, and nutrients to the heart. A stent can be inserted to widen the artery, but these devices must be closely monitored to ensure that they do not re-narrow, a common complication called restenosis.
McGowan Institute for Regenerative Medicine affiliated faculty member Youngjae Chun, PhD, associate professor of industrial engineering and bioengineering at the University of Pittsburgh’s Swanson School of Engineering, will lead a study to develop an electronic stent that can be implanted in a minimally invasive procedure and measure significant physiological changes with the development of restenosis. The device will provide real-time monitoring to help prevent subsequent heart attack or stroke.
The project, funded by the National Institutes of Health, is in collaboration with W. Hong Yeo, PhD, assistant professor of mechanical engineering at Georgia Tech, and McGowan Institute affiliated faculty member John Pacella, MD, cardiologist at UPMC, who also holds a secondary appointment in bioengineering.
This work is a continuation of the group’s 2019 Innovative Project Award from the American Heart Association.
“Though similar devices already exist, they are typically bulkier and do not work as effectively with the growth of artery tissue,” said Dr. Chun.
This new device has an ultra-low profile sensor, which allows it to work without a battery and wirelessly monitor the restenosis progress. They believe that this device can easily integrate with commercially available stents without disturbing its functionality. With this design, users will be able to see real-time data on a smart device, rather than scheduling endless follow-up visits to the doctor.
The group will use computational modeling and calculation to carefully design the device, and then it will then be fabricated using a novel nanoscale printing technique. Once it is developed, they will evaluate the design in vitro to determine its functionality with a stent.
Previous sensors are only able to monitor stents for a few days or weeks after the implant procedure, but their design will be able to continuously monitor, providing a unique, long-term solution.
Dr. Chun said, “This kind of translational research is a strength of the University of Pittsburgh, and we hope that this technology can eventually be expanded to other endovascular devices where specific physiological changes in our vascular system are a factor in the remodeling process.”
Illustration: Surgeons can widen arteries and implant a stent during a balloon angioplasty procedure. The stent needs to be closely monitored to make sure it does not re-narrow. Credit: Shutterstock.
For COVID-19, Immune System Can Be ‘A Hero or A Villain’
When a person contracts COVID-19, or any other respiratory virus, the immune system springs into action. Body aches and fever are two signs the body is trying to slow the infection and fight off the virus. The problem is that sometimes, the body doesn’t know when to stop.
“In respiratory infection, the immune response can be the hero and the villain,” said McGowan Institute for Regenerative Medicine affiliated faculty member Jason Shoemaker, PhD. “A reasonable immune response should control the infection while protecting our body, but aggressive immune responses can often lead to increased tissue damage or even death during infection.”
An overly aggressive immune response can make recovery from COVID-19 riskier and cause long-lasting damage, such as diminished lung capacity and increased tissue damage, or even death.
Why are some bodies able to fight off the virus without causing damage to healthy tissue when others cannot? Dr. Shoemaker, assistant professor of chemical engineering in the Swanson School of Engineering, and his team are determined to find out. The team includes University of Pittsburgh researchers Penelope Morel, MD, professor of immunology, and James Faeder, PhD, associate professor of computational and systems biology.
“It is clear that increased disease severity leads to permanent damage of the lung tissue, which in very extreme cases has necessitated lung transplantation,” said Dr. Morel.
“Even patients with relatively mild disease may take months to fully recover and may experience symptoms such as reduced lung function, chronic fatigue, clotting disorders and more,” she continued. “Thus, it is important to understand how the healing process may be dysregulated in COVID-19 patients.”
Using agent-based modeling, they are learning how the virus behaves by mapping the body’s immune response.
“Agent-based modeling is a modeling method more akin to video game design than most models in engineering,” explained Dr. Shoemaker. “It is based on choice: in this situation, based on what we know, what action would the cell be most likely to take?”
By following the virus’s path through the body, the team is creating a detailed simulation that can uncover the biomarkers and signs that may predict an overly aggressive immune response. That would allow doctors to treat those patients accordingly.
“Our modeling can help doctors determine when to use the drugs we already have on the market: We want an immune response that is strong enough to clear the virus, but we want to be able to suppress the immune system if necessary before it begins to cause damage,” explained Dr. Shoemaker. “The timing of drug intervention is one of the most difficult parts in treating disease, but engineering is great at working with that kind of precise timing.”
Dr. Shoemaker recently received a National Science Foundation CAREER Award for this work.
From mapping the virus’s actions in individual cells to understanding the effects on the lungs as a whole, each member of the team is working to piece together this puzzle, creating a comprehensive model that ideally will predict how the virus that causes COVID-19, SARS-CoV-2, infects the lungs, and the damage it leaves behind.
In addition to the Pitt team’s modelling work, the group is collaborating with an international team led by Indiana University researcher Paul Macklin, PhD, associate professor of intelligent systems engineering, to create larger-scale models that could inform pharmaceutical interventions. The initial agent-based model has been developed in close collaboration with James Glazier, PhD, professor of physics, also at Indiana University.
“Our work will enable us to identify the best means of controlling the infection by either regulating the immune system itself or by identifying new human proteins to consider for drug targeting,” said Dr. Shoemaker. “This virus is going to be with us for some time, so it’s important that we understand how to help our bodies react to it in the best possible way.”
Getting the Full Scope
A neural interface — or brain-computer interface — is a device that uses electrical stimulation to induce changes in brain activity. Among other applications, its positive effects are being leveraged to rehabilitate neurological conditions, such as Parkinson’s disease.
These implantable devices have recently been in the headlines with Elon Musk’s new company, Neuralink, which seeks to make tools to treat brain diseases and eventually expand to human enhancement.
But according to experts, there is still much to learn about this pioneering technology and how it affects this vital organ. Much like the ocean or the universe, there are many undiscovered functions of the brain, and the McGowan Institute for Regenerative Medicine affiliated faculty member Takashi Kozai, PhD, and University of Pittsburgh’s Alberto Vazquez, PhD, seek to further explore this scientific frontier.
The brain is composed of a variety of cells, each with its own set of properties. While neurons are the most well-known, there are many supportive cells that are necessary for proper function. Dr. Kozai will use a $3,051,391 BRAIN Initiative R01 award from the National Institutes of Health to investigate what drives neural interfaces’ beneficial effects by studying how stimulation affects non-neuronal brain cells over time.
“There isn’t much research on how electrical stimulation affects cells other than neurons in the brain, and there is mounting evidence that suggests glia and the vasculature play a large role,” said Dr. Kozai, assistant professor of bioengineering at Pitt’s Swanson School of Engineering.
“Our understanding of the brain has been limited by our focus on neurons, and this creates a significant barrier to the design of new and improved clinical applications and the interpretation of stimulation-induced effects in basic science research,” he continued.
Many neurological diseases are actually a dysfunction of non-neuronal cells or the neurovasculature: multiple sclerosis is a disease of the oligodendrocytes; Alzheimer’s disease may start with neurovasculature dysfunction; stroke is a neurovascular injury that impacts neurological function; and glioma is a cancer of the glia.
The problem with studying this part of the brain is that the slow, dim signals of non-neuronal cells are much more difficult to detect than the vibrant electrical activity of neurons. Dr. Kozai’s solution is to use two-photon microscopy and optogenetics to investigate the inner workings of the brain.
“In single photon microscopy, you take a high energy photon and collide it with an electron, which pushes it to the next orbital, and as it falls down, it releases a fluorescent light,” Dr. Kozai explained. “The difficulty with this approach is the amount of out-of-focus fluorescence that saturates the image.
“With two-photon microscopy, you take two photons with half the energy and collide them simultaneously with the electron,” he continued. “This approach eliminates out-of-plane fluorescence and allows you to image deeper into the tissue.”
Imaging deeper into brain tissue is important because the first layer of the cortex does not contain neurons, and researchers must penetrate further to see how neurons and non-neuronal cells interact. With modern technology, the team can insert normal — or even modified — green fluorescent proteins that report cellular changes, such as calcium or neurotransmitter levels, into specific cell types and monitor their activity over time.
Dr. Kozai’s BIONIC Lab, will use this project to build on their expertise in neural interface technology. In January 2020, he received a $1,652,844 NIH award to develop a wireless in vivo stimulation technology that will enable precise neural circuit probing while minimizing tissue damage. He also received a 2020 CAREER award from the National Science Foundation to advance neural interface design by studying how different types of stimulation impact neuronal subtype activity.
“We’re grateful for the opportunity to apply our experience and expertise to understanding some long-standing fundamental science questions at the brain-computer interface,” said Dr. Kozai. “These grants together give us an opportunity to ask some fundamental questions regarding the biological underpinning of neurostimulation and neuromodulation-related activity for a diverse set of cells in the brain.”
“In turn, this allows us to integrate how these diverse cell activities fit together in a system-of-systems engineering perspective,” he continued. “The long-ranged vision is to identify new relationships between these different cell types and how to synergistically modulate them to improve therapies for neurological diseases and brain injuries. Ultimately, this requires researchers from a diverse set of cultural and socioeconomic backgrounds.”
Illustration: Electrical stimulation through microelectrode arrays (white) in the brain activate microglia (green), pericyte (red; cells that are directly infected by SARS-Cov-2), and blood vessel activity (blue) to modulate neural activity. Image by Steven Wellman.
Rapid Blood Test Could Detect Brain Injury in Minutes
A blood protein test could detect the severity of head trauma in under 15 minutes, according to research published recently in the Journal of Neurotrauma.
By showing that glial fibrillary acidic protein (GFAP) can accurately determine the severity of a brain injury through a blood test, the research team working on this study, led by author David Okonkwo, MD, PhD, director of the Neurotrauma Clinical Trials Center at UPMC, professor of neurological surgery at the University of Pittsburgh School of Medicine, and affiliated faculty member of the McGowan Institute for Regenerative Medicine, advanced the development of a point-of-care testing device designed to help clinicians assess traumatic brain injury (TBI) in minutes.
For the rapid test, the vision included using a hand-held device with a cartridge that would measure GFAP in a patient’s blood. Researchers at Abbott Laboratories, a global health care company, will need to finalize the test for the i-STAT device, which already is used by the military and health care providers around the world to perform several common blood tests within minutes. The blood test would reveal a patient’s GFAP level.
“This would eliminate guesswork in diagnosing TBIs and learn whether a person needs further treatment,” said Dr. Okonkwo. “Whether you’re testing a soldier injured in combat or testing a patient in a small rural hospital with limited resources, health care providers could have critical information they need—in minutes—to treat each patient’s brain injury.”
For this study, which expanded upon previous GFAP findings, researchers enrolled 1,497 people who sought care at one of the 18 Transforming Research and Clinical Knowledge in TBI (TRACK-TBI) level 1 trauma centers nationwide over four years. GFAP is a Food and Drug Administration-approved marker for ruling out whether a patient needs a head computed tomography (CT) scan within 12 hours after a mild TBI.
For years, scientists have studied blood tests involving GFAP. They also have studied a similar protein called S100B. Both proteins are released in the bloodstream in response to specific injuries, including TBI. But this study showed that GFAP substantially outperformed S100B as a TBI diagnostic marker.
“Knowing this protein can show the severity of a TBI through a simple blood test is promising when considering we can use a device that already is in widespread use in hospitals, doctors’ offices and urgent care facilities. All we would need to do is add an extra cartridge to the device to analyze blood for the GFAP protein,” said Dr. Okonkwo. He estimates this device could potentially decrease unnecessary CT scans by 20% or more, saving nearly $100 million in medical expenses annually.
With support from the U.S. Army Medical Materiel Research and Development Command’s U.S. Army Medical Materiel Development Activity, Abbott Point-of-Care and TRACK-TBI have begun a pivotal, FDA-regulated trial to validate the i-STAT to evaluate the effectiveness of Abbott’s point-of-care blood test technology, using whole blood sample type.
This published study is a collaboration between the National Institutes of Health (NIH), the U.S. Department of Defense, the TRACK-TBI consortium and Abbott Laboratories. This work was supported with funding from the NIH National Institute of Neurological Disorders and the U.S. Department of Defense.
Team Receives $1M NSF Award to Create At-Home Glaucoma Monitoring Device
Diabetic patients monitor their blood glucose throughout the day, watching for peaks and valleys. Just taking a sample once during a visit to the doctor’s office would not give a clear picture of whether the patient’s diabetes is under control. The same is true of glaucoma patients, whose intraocular pressure (IOP), or pressure within the eye, is too high.
IOP varies throughout the day, but there isn’t yet an easy way to monitor changes at home that would provide proven, reliable readings, making it difficult for doctors to monitor the effectiveness of treatment.
Piervincenzo Rizzo, PhD, professor of civil and environmental engineering at the University of Pittsburgh’s Swanson School of Engineering, is leading a project that will help glaucoma patients monitor their intraocular pressure (IOP) at home, giving them and their doctors a clearer picture of eye health. McGowan Institute for Regenerative Medicine affiliated faculty members Ian Sigal, PhD, Associate Professor, Director of the Laboratory of Ocular Biomechanics in the Department of Ophthalmology at the University of Pittsburgh School of Medicine, and Ian Conner, MD, PhD, Assistant Professor of Ophthalmology and Bioengineering at the University of Pittsburgh, are members of the research team.
The project, titled “Managing Glaucoma in the Digital Age: A New Tonometer to Connect Patients to their Caregivers,” recently received $1,099,984 from the National Science Foundation. Work will begin October 1, 2020, and last four years.
“We understand that intraocular pressure can have a pretty wide range throughout the day but have very few ways to assess this critical variable outside of the clinic,” explained Dr. Conner, Director of UPMC’s Glaucoma Service. “This technology really has a lot of potential to enable non-clinicians, and even patients themselves, to reliably assess intraocular pressure, which will allow their doctors to better tailor their treatments.
The proposed device would use a cylinder containing an array of particles that, when pressed against the closed eyelid, will send an acoustic wave into the eye and wait for it to bounce back. The properties of the returning wave give the device information about the pressure inside the eye.
“We’re proposing to use a special family of acoustic waves that can interact with the eye, bouncing back like an echo,” said Dr. Rizzo. “It’s like shouting into a small room versus a large one. The properties of the echo depend on the properties of the room.”
Dr. Rizzo’s team also includes Sam Dickerson, PhD, assistant professor of electrical and computer engineering at the Swanson School, and Robert Handzel, MD, in Pitt’s Department of Ophthalmology.
An Approach to the Protein Aggregation Problem in Therapeutic Bioprocessing
A bunched-up bundle of a protein-based biologic is practically useless. Unfortunately, protein aggregation creates a common problem in the bioprocessing of many therapeutics. Addressing this issue will probably depend on analyzing the problem in new ways, which could trigger changes in various parts of the process.
Recently, McGowan Institute for Regenerative Medicine affiliated faculty member Wilson Meng, PhD, professor of pharmaceutics at Duquesne University’s School of Pharmacy, and graduate research assistant Ngoc Pham reviewed this problem and potential solutions. Mike May, PhD, reported on their work for Genetic Engineering & Biotechnology News.
When asked to pick out the most important finding from this study, Dr. Meng says, “There is a need to break from conventional formulation and development approaches. Specifically, applying linear models trained using low-concentration formulations to high protein-concentration formulations would likely yield invalid results.”
Instead, scientists need more advanced approaches, Dr. Meng believes. “Researchers should invest in tailoring predictive modeling and analytical approaches to a given protein biotherapeutic,” he explains. “Each new protein entity deserves its own pre-formulation and formulation solutions.”
So, the processes might require more customization that matches the needs of a specific biotherapeutic to avoid aggregation.
Even more opportunities for advances lies ahead. For example, the method of preventing protein aggregation could vary in different bioprocessing systems. As Dr. Meng says, “Another area of opportunity is that there is a knowledge gap in controlling protein aggregation in cell-free synthetic manufacturing systems.”
As Dr. Meng and Mr. Pham described the goal of their study: “The purpose is to stimulate new dialogs that would bridge the interface between physical characterizations of protein aggregates in biotherapeutics and the functional attributes of the immune system.”
So, to keep biotherapeutics apart, scientists need to get together.
Catalyzing an Improved Arterial Bypass Graft
Just as a climbing plant needs the right trellis to thrive, a small-diameter tissue-engineered vascular graft (TEVG) needs the right scaffold to transform seeded cells into a native-like artery that can save a life.
A team led by McGowan Institute for Regenerative Medicine affiliated faculty member David Vorp, PhD, Associate Dean for Research, Swanson School of Engineering, and the John A. Swanson Professor of Bioengineering, University of Pittsburgh, received a $1.1M award from the National Institutes of Health to optimize this emerging technology for cardiovascular disease. They will examine the best combination(s) of active “payload” and scaffold to develop a feasible alternative to the decades-old practice of using vessels harvested from a patient’s own chest or leg.
Coronary heart disease – a worldwide leading cause of death – damages arteries that carry a vital supply of blood, oxygen, and nutrients to the heart. Surgeons typically replace damaged vessels with healthy autologous ones that are harvested from a different part of the patient’s body, but according to Dr. Vorp, they are not an ideal substitution.
“Autologous vessels are not ideal in that they are limited in number and/or are not naturally designed to function as an artery,” he said. “They have been the gold standard in bypass grafts, but in recent years, companies have begun clinical testing on TEVGs developed in research labs like ours.”
Dr. Vorp’s team has developed a TEVG based on the well-known regenerative power of mesenchymal stem cells (MSCs), which both prevent blood from clotting on the implanted TEVG and recruit host immune cells that participate in the regeneration process. MSCs are adult stem cells most often derived from a patient’s bone marrow.
A successful TEVG will grow and remodel into a native-like artery. It consists of a scaffold that provides a framework for seeded cells, which when given environmental cues, will promote tissue regeneration. In this project, Dr. Vorp and his collaborators will examine a variety of successful “payloads” and scaffolds to determine which combinations work best.
For the payload, the group will study a cell-based and cell-free approach using both MSCs and – for the cell-free approach – certain immunoregulatory factors that the MSCs secrete.
“We believe that the regulatory pathway for a cell-free configuration would be faster if it is shown to be as effective as a cell-based approach,” Dr. Vorp said.
They will assess each feasible combination of payload and biodegradable scaffold, which will be made from materials in the polyurethane and silk families.
“Our previous work has focused on the ability of some of our payload and scaffold combinations to remodel into a successful TEVG when implanted as an aortic replacement graft in rats,” said Dr. Vorp. “This NIH Catalyze grant will now allow us to more rigorously optimize the grafts in the small animal model to narrow down the number of combinations to be tested in a large animal model.”
Finding the best combination(s) of payload and scaffold is only the first step of this project. It is part of a two-phase Catalyze grant from the NIH’s National Heart Lung and Blood Institute, which includes a one-year R61 grant in which the team must achieve the necessary milestones to be eligible to transition to the two-year R33 award.
In the second part of the project, the group will use the R33 award to address the manufacturability and other clinical translational aspects of a TEVG, including large animal testing of the best configuration(s).
“We will work with ‘accelerator partners,’ including RoosterBio, Inc. and Pitt’s Clinical & Translational Sciences Institute, as well as regulatory consultants to begin addressing manufacturability for clinical translation,” Dr. Vorp said.
Though there are many advantages to TEVGs, the technology also has its challenges. The researchers hope that finding an optimal configuration will decrease the chance of stenosis, a common complication where the vessel narrows and limits blood flow.
The goal of this award is to find a design that can advance to the clinical phase of development and eventually reach the market as a better-quality graft for bypass surgery.
Dr. Vorp also holds secondary appointments in the Departments of Cardiothoracic Surgery, Surgery, and the Clinical & Translational Sciences Institute at the University of Pittsburgh. He also serves as a Co-Director of the Center for Medical Innovation and the Director of the Vascular Bioengineering Laboratory.
Dr. Dennis McNamara to Lead Cardiol Therapeutics Phase II/III COVID-19 Trial
Cardiol Therapeutics Inc., a clinical-stage biotechnology company focused on developing innovative therapies for inflammatory heart disease, received the U.S. Food and Drug Administration (FDA) approval for its Investigational New Drug application to commence a Phase II/III, double-blind, placebo-controlled clinical trial investigating the efficacy and safety of CardiolRx™, a pharmaceutically produced extra strength cannabidiol formulation, in 422 hospitalized COVID-19 patients with a prior history of, or risk factors for, cardiovascular disease (CVD). The trial will take place at major centers in the United States, where the prevalence of COVID-19 remains high. McGowan Institute for Regenerative Medicine affiliated faculty member Dennis McNamara, MD, Professor of Medicine, University of Pittsburgh, and the Director of the Heart Failure/Transplantation Program at the University of Pittsburgh Medical Center, is Chair of the Steering Committee for the trial.
Dr. McNamara commented: “As a Steering Committee, we are excited about the potential for this study to contribute valuable new information about the role of anti-inflammatory agents in the management of COVID-19. Now that approval from the FDA has been granted, we are anxious to get underway so that we can investigate the impact of CardiolRx in this very important disease process.”
Patients with COVID-19 primarily present with respiratory symptoms which can progress to bilateral pneumonia and serious pulmonary complications. It is now recognized that the impact of COVID-19 is not limited to the pulmonary system. Individuals with pre-existing CVD or who have risk factors for CVD (such as diabetes, hypertension, obesity, abnormal serum lipids, or age greater than 64) are at significantly greater risk of developing serious disease from COVID-19 and experience greater morbidity. Moreover, such COVID-19 patients are at significant risk of developing cardiovascular complications (such as acute myocardial infarction, cardiac arrhythmias, myocarditis, stroke, and heart failure) during the course of their illness, and which are frequently fatal, with an estimated 30 – 40% of patients who die from COVID-19 doing so from cardiovascular complications. A strategy to prevent or limit the number or severity of these cardiovascular complications is likely to considerably improve outcomes from this disease.
Cardiol’s Phase II/III trial has been designed to assess the efficacy, safety, and tolerability of CardiolRx™ in preventing cardiovascular complications in hospitalized patients, with a confirmed diagnosis of COVID-19 within the previous 24 hours, and who have pre-existing CVD and/or significant risk factors for CVD. The composite primary efficacy endpoint will be the difference between the active and placebo groups in the percentage of patients who develop, during the first twenty-eight days following randomization and first dose of study medication, a composite endpoint consisting of one or more of several common outcomes in this patient population, including all-cause mortality, requirement for ICU admission and/or ventilatory support, as well as cardiovascular complications, including the development of heart failure, acute myocardial infarction, myocarditis, stroke, or new sustained or symptomatic arrhythmia.
The rationale for using cannabidiol to treat patients with COVID-19 is based on extensive pre-clinical investigations by Cardiol and others in models of cardiovascular inflammation which have demonstrated that CBD has impressive anti-inflammatory and anti-fibrotic activity, as well as anti-ischemic, and anti-arrhythmic action, and that it improves myocardial function in models of heart failure. In pre-clinical models of cardiac injury, cannabidiol was shown to be cardio-protective by reducing cardiac hypertrophy, fibrosis, and the production of certain re-modelling markers, such as cardiac B-type Natriuretic Peptide (BNP), which is typically elevated in patients with heart failure. These data were accepted for presentation at the American College of Cardiology’s 69th Annual Scientific Session held virtually on March 28 – 30, 2020.
Study Shows How COVID-19 Could Lead to Runaway Inflammation
A study from the University of Pittsburgh School of Medicine and Cedars-Sinai addresses a mystery first raised in March: Why do some people with COVID-19 develop severe inflammation? The research shows how the molecular structure and sequence of the SARS-CoV-2 spike protein—part of the virus that causes COVID-19—could be behind the inflammatory syndrome cropping up in infected patients.
The study, published in the Proceedings of the National Academy of Sciences, uses computational modeling to zero in on a part of the SARS-CoV-2 spike protein that may act as a “superantigen,” kicking the immune system into overdrive as in toxic shock syndrome—a rare, life-threatening complication of bacterial infections. McGowan Institute for Regenerative Medicine affiliated faculty member Ivet Bahar, PhD, Distinguished Professor, the John K. Vries Chair, and the Founding Chair in the Department of Computational & Systems Biology at the University of Pittsburgh’s School of Medicine, is a co-senior author on the study.
Symptoms of a newly identified condition in pediatric COVID-19 patients, known as Multisystem Inflammatory Syndrome in Children (MIS-C), include persistent fever and severe inflammation that can affect a host of bodily systems. While rare, the syndrome can be serious or even fatal.
The first reports of this condition coming out of Europe caught the attention of study co-senior author Moshe Arditi, MD, director of the Pediatric Infectious Diseases and Immunology Division at Cedars-Sinai and an expert on another pediatric inflammatory disease—Kawasaki disease.
Dr. Arditi contacted his long-time collaborator, Dr. Bahar, and the two started searching for features of the SARS-CoV-2 virus that might be responsible for MIS-C.
Dr. Bahar and her team created a computer model of the interaction between the SARS-CoV-2 viral spike protein and the receptors on human T cells, the foot soldiers of the immune system. Under normal circumstances, T cells help the body fight off infection, but when these cells are activated in abnormally large quantities, as is the case with superantigens, they produce massive amounts of inflammatory cytokines—small proteins involved in immune system signaling—in what’s known as a “cytokine storm.”
Using this computer model, the team was able to see that a specific region on the spike protein with superantigenic features interacts with T cells. Then, they compared this region to a bacterial protein that causes toxic shock syndrome and found striking similarities in both sequence and structure. Importantly, the proposed SARS-CoV-2 superantigen showed a high affinity for binding T cell receptors—the first step toward touching off a runaway immune response.
“Everything came one after another, each time a huge surprise. The pieces of the puzzle ended up fitting extremely well,” said Dr. Bahar.
By finding protein-level similarities between SARS-CoV-2 and the bacterial structure that causes toxic shock syndrome, the researchers said they may have opened up new avenues for treating not only MIS-C patients, but also adults with COVID-19 infection experiencing cytokine storm.
The researchers also collaborated with scientists studying adult COVID-19 patients in Germany and found that those who experienced severe symptoms had a T cell response similar to what is seen in people exposed to superantigens and very different from the T cell response in patients who had only mild symptoms.
“Our research finally begins to unravel the potential mechanisms involved and raises the possibility that therapeutic options for toxic shock syndrome, such as intravenous immunoglobulin and steroids, may be effective for managing and treating MIS-C in children and hyperinflammation in adult coronavirus patients,” said Dr. Arditi, professor of pediatrics and biomedical sciences at Cedars-Sinai.
Drs. Arditi and Bahar’s labs are now using the ideas generated by this study to search for and test antibodies specific to the SARS-CoV-2 superantigen, with the goal of developing therapies that specifically address MIS-C and cytokine storm in COVID-19 patients.
AWARDS AND RECOGNITION
In Tribute: Ed Kastriba
We sadly share the news of the passing of Ed Kastriba, pre-awards coordinator for the McGowan Institute. We depended on Ed to expertly manage our grant submissions, and he always delivered with care and precision. Ed’s service to the Institute and to the advancement of regenerative medicine will be long remembered and appreciated.
Please keep Ed and his family in your prayers.
Dr. Sanjeev Shroff Inducted as Fellow of the International Academy of Medical and Biological Engineering
McGowan Institute for Regenerative Medicine faculty member Sanjeev Shroff, PhD, Distinguished Professor and Gerald E. McGinnis Chair of Bioengineering at the University of Pittsburgh, was elected as a Fellow of the International Academy of Medical and Biological Engineering (IAMBE). The virtual induction ceremony was held in conjunction with the Carnegie Mellon Forum on Biomedical Engineering on September 18, 2020.
This competitive election is in recognition of distinguished contributions to and leadership in the field of medical and biological engineering on an international level. Presently, there are only around 200 living Fellows of the Academy throughout the world.
Dr. Shroff was selected for his significant research contributions to cardiovascular engineering and bioengineering education. His research in Pitt’s Swanson School of Engineering has two focus areas: (1) regulation of cardiac muscle contraction by changes in cardiac proteins and their chemical modifications and (2) the role of vascular stiffness in cardiovascular function and potential therapeutic applications of vascular stiffness-modifying drugs and/or hormones.
His research efforts have been continuously funded by the National Institutes of Health since 1986, with additional support from other funding agencies such as American Heart Association, National Science Foundation, and industry.
“Elucidating the links between the overall cardiovascular mechanical function and underlying cellular and molecular processes has been the focus of my research, and I am honored that this work has been recognized by IAMBE,” said Dr. Shroff. “I have had the good fortune to collaborate with and learn from talented biomedical scientists and clinicians and I would like to share this honor with them.”
In addition to his leadership in research, Dr. Shroff also has a strong record in teaching and mentorship. He has mentored 37 pre- and post-doctoral students and led the effort to establish the department’s Cardiovascular Bioengineering Training Program, which has been supported by an NIH training grant (T32) since its launch in 2005.
Trained as an electrical engineer (B.Tech., Indian Institute of Technology, Kanpur, India and M.Eng., McMaster University, Canada), Dr. Shroff obtained his doctoral degree in Bioengineering from the University of Pennsylvania. Prior to joining the University of Pittsburgh in April 2000, Dr. Shroff was a faculty member at the University of Chicago for 17 years in the Department of Medicine (Cardiology Section).
Dr. Shroff was the recipient of the Established Investigator Award from the AHA (1986-1991) and was elected as a Fellow of the American Physiological Society (1988), Fellow of the American Institute for Medical and Biological Engineering (1999), and Fellow of Biomedical Engineering Society (2007). Recognized by his colleagues and peers as a consummate teacher and mentor, he received the Carnegie Science Center Award for Excellence (University Educator) in 2007, the Swanson School of Engineering’s Outstanding Educator Award in 2010, and University of Pittsburgh Chancellor’s Distinguished Teaching Award in 2011.
He has mentored 37 students (15 post-doctoral and 22 graduate/pre-doctoral), most of whom are pursuing independent research careers in academia or industry. He has been serving as the Principal Investigator on a NIH-NHLBI pre-doctoral T32 training grant (Cardiovascular Bioengineering Training Program) since 2005 and on the Coulter Translational Research Partnership II grant since 2013. In 2012, Dr. Shroff was named the Distinguished Professor at the University of Pittsburgh, a designation that constitutes the highest honor that the University can accord a member of the professoriate.
Congratulations, Dr. Shroff!
Dr. Ora Weisz Named 2020 Fellow of ASCB
The American Society for Cell Biology (ASCB) Council has named its 2020 cohort of Fellows, ASCB members selected for their lifetime achievements in advancing cell biology. McGowan Institute for Regenerative Medicine affiliated faculty member Ora Weisz, PhD, was one of 18 members who were chosen for their outstanding contributions to the field of cell biology and to the community of cell biologists through their service to the Society.
Dr. Weisz is a Professor in the Departments of Medicine (Renal-Electrolyte Division), Cell Biology, and Clinical and Translational Science at the University of Pittsburgh. She is also the Vice Chair of Faculty Development in the Department of Medicine, the Associate Dean for Faculty Development in Pitt’s School of Medicine, and Assistant Vice Chancellor for Faculty Excellence, Health Sciences.
Dr. Weisz’s research interest focuses broadly on understanding how membrane traffic in proximal tubule cells responds to physiologic cues to maintain kidney function. Her team is unraveling the mechanisms by which proximal tubule cells in the kidney alter their endocytic and ion transport capacity in response to changes in tubular flow and the accompanying fluid shear stress. These studies have direct implications for the understanding and treatment of genetic and other disorders that result in tubular proteinuria and eventually lead to kidney failure, including Lowe syndrome, Dent disease, and sickle cell disease.
The ASCB Fellows program is committed to recognizing the breadth and diversity of the Society’s membership. Toward this end, Fellows are nominated by their peers or through self-nomination, followed by evaluation and selection by a Fellows Nomination Review Committee. The list of selected Fellow nominees is reviewed and approved by the ASCB Council. The ASCB Fellows will be formally recognized at Cell Bio Virtual 2020–An Online ASCB|EMBO Meeting in December 2020.
Congratulations, Dr. Weisz!
Dr. Derek Angus Named Associate Vice Chancellor for Health Care Innovation
McGowan Institute for Regenerative Medicine affiliated faculty member Derek Angus, MD, MPH, has been named University of Pittsburgh’s inaugural associate vice chancellor for health care innovation, a role that will complement his recent appointment as UPMC’s chief health care innovation officer and foster more strategic linkages between the two organizations to enable learning health systems.
In his new University role, Dr. Angus will work to stimulate the fusion of multiple disciplines and skills, blending expertise in clinical care delivery with organization science, decision psychology, machine learning, Bayesian trial designs, causal inference, implementation science and behavioral economics, among others.
Dr. Angus’ mandate is to enable learning while doing—to make smarter, faster decisions and create better integration across all of the translational, clinical, and health services and health policy domains on campus. In doing so, Dr. Angus will be supporting the entire health sciences community in working together more effectively and in concert with UPMC.
Dr. Angus will give equal attention to creative disruption in health sciences education. Progress in health care innovation requires the development of leaders and teams with considerable range across disciplines that have not necessarily been emphasized and have rarely been united in the same person or team. Thus, Dr. Angus will also be identifying opportunities to think about curricular innovations and novel training programs across the health sciences.
Dr. Angus is ideally positioned for his new roles. He is an internationally renowned and highly prolific scientist who has developed and led many successful multidisciplinary collaborations of basic scientists, clinicians, data scientists, economists, and behavioral and social scientists. In recent years, he has been a leader in developing and evaluating approaches that facilitate smarter decision-making and faster learning in health care, including novel Bayesian adaptive trial designs, the application of machine learning to large-scale data, and the use of behavioral economics and decision psychology to support optimal decision-making.
Dr. Angus is a Fellow of the Royal College of Physicians of the United Kingdom and has received multiple national and international honors. He completed medical school and internal medicine training at the University of Glasgow and affiliated teaching hospitals, and he completed a fellowship in critical care medicine and his MPH in health services administration at Pitt.
Congratulations, Dr. Angus!
Regenerative Medicine Podcast Update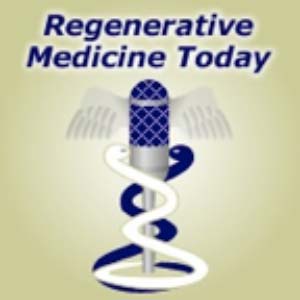
The Regenerative Medicine Podcasts remain a popular web destination. Informative and entertaining, these are the most recent interviews:
#214 –– Dr. Fabrisia Ambrosio discusses regenerative rehabilitation and the Alliance for Regenerative Rehabilitation Research and Training.
Visit www.regenerativemedicinetoday.com to keep abreast of the new interviews.
PUBLICATION OF THE MONTH
Author: David O. Okonkwo, Ross C. Puffer, Ava M. Puccio, Esther L. Yuh, John K. Yue, Ramon Diaz-Arrastia, Frederick K. Korley, Kevin K. W. Wang, Xiaoying Sun, Sabrina R. Taylor, Pratik Mukherjee, Amy J. Markowitz, Sonia Jain, Geoffrey T. Manley, The Transforming Research and Clinical Knowledge in Traumatic Brain Injury (TRACK-TBI) Investigators
Title: Point-of-Care Platform Blood Biomarker Testing of Glial Fibrillary Acidic Protein versus S100 Calcium-Binding Protein B for Prediction of Traumatic Brain Injuries: A Transforming Research and Clinical Knowledge in Traumatic Brain Injury Study
Summary: Glial fibrillary acidic protein (GFAP) is cleared by the Food and Drug Administration (FDA) to determine need for head computed tomography (CT) within 12 h after mild traumatic brain injury (TBI) (Glasgow Coma Score [GCS] 13–15); S100 calcium-binding protein B (S100B) serves this function in Europe. This phase 1 biomarker cohort analysis of the multi-center, observational Transforming Research and Clinical Knowledge in Traumatic Brain Injury (TRACK-TBI) study compares GFAP’s diagnostic performance, measured on a rapid point-of-care platform, against protein S100B to predict intracranial abnormalities on CT within 24 h post-injury across the spectrum of TBI (GCS 3–15). Head CT scan performed in TBI subjects and blood was collected for all consenting subjects presenting to 18 United States level 1 trauma centers. Plasma was analyzed on a point-of-care device prototype assay for GFAP and serum was analyzed for S100B. In 1359 patients with TBI (GCS 3–15), mean (standard deviation [SD]) age = 40.1 (17.0) years; 68% were male. Plasma GFAP levels were significantly higher in CT+ TBI subjects (median = 1358 pg/mL, interquartile range [IQR]: 472–3803) than in CT- TBI subjects (median = 116 pg/mL, IQR: 26–397) or orthopedic trauma controls (n = 122; median = 13 pg/mL, IQR: 7–20), p < 0.001. Serum S100B levels were likewise higher in CT+ TBI subjects (median = 0.17 μg/L, IQR: 0.09–0.38) than in CT- TBI subjects (median = 0.10 μg/L, IQR: 0.06–0.18), p < 0.001. Receiver operating characteristic curves were generated for prediction of intracranial injury on admission CT scan; area under the curve (AUC) for GFAP was significantly higher than for S100B in the same cohort (GFAP AUC – 0.85, 95% confidence interval [CI] 0.83–0.87; S100B AUC – 0.67, 95% CI 0.64-0.70; p < 0.001). GFAP, measured on a point-of-care platform prototype assay, has high discriminative ability to predict intracranial abnormalities on CT scan in patients with TBI across the full injury spectrum of GCS 3–15 through 24 h post-injury. GFAP substantially outperforms S100B.
Source: Journal of Neurotrauma. 2020 Sep 14. doi: 10.1089/neu.2020.7140. Online ahead of print.
GRANT OF THE MONTH
PIs: D. Lansing Taylor, Jaideep Behari, and Alejandro Soto-Guttierrez
Title: A Vascularized Patient-Derived iPSC Liver Acinus Microphysiological System as an Innovative Precision Medicine Platform for Optimizing Clinical Trial Design for Nonalcoholic Fatty Liver Disease
Description: A vascularized patient-derived iPSC liver acinus microphysiology system (vLAMPS) is an innovative precision medicine platform for optimizing clinical trial design for nonalcoholic fatty liver disease (NAFLD). Non-alcoholic fatty liver disease (NAFLD) is a major health crisis with no approved therapeutics and many failures in the clinic. The prevalence of NAFLD is estimated to increase from 25% of the US population in 2015 (~83 million) to over 100 million by 2030, accompanied by an increase in nonalcoholic steatohepatitis (NASH), the progressive form of the disease, that can lead to cirrhosis with liver failure and hepatocellular carcinoma (HCC). Despite its public health importance, there is currently no FDA-approved therapy for any stage of NAFLD. NAFLD/NASH is a complex heterogeneous disorder involving multiple molecular pathways. Development of efficacious pharmacotherapy has been hampered by the limited utility of preclinical drug testing models. Simple cell culture and animal models do not recapitulate the spectrum of NASH phenotypes in humans. Highlighting these species differences, knock-in murine models with the high-risk NASH-associated genetic polymorphism, PNPLA3 I148M, develop hepatic steatosis but do not recapitulate the progressive disease seen in humans. Additionally, heterogeneity in risk of progression of NASH, individual genetic variations modulating risk of fibrosis progression, and presence of NAFLD-associated metabolic comorbidities such as Type 2 diabetes mellitus (T2DM), adds additional complexity. We will implement the vLAMPS to initially characterize both a “normal” and a NAFLD/NASH vLAMPS generated from primary human liver cells (hepatocytes, liver sinusoidal endothelial cells, stellate and Kupffer cells) and then reproduce the results with induced pluripotent stem cells (iPSCs). We will ultimately generate patient-specific iPSCs of the four cell types from patients in our NAFLD clinic to create patient-specific vLAMPS. We will test two cohorts: 1) patients with the PNPLA3 I 148M variant and 2) patients with the wild-type PNPLA3 to identify the patients who respond to two NAFLD drugs that have or are now going through clinical trials and two control drugs. Importantly, this paradigm circumvents the conundrum of high-risk patients being enrolled in large prolonged studies with a high likelihood of failure being simultaneously disqualified from other potentially beneficial studies/treatments. This approach will prove transformational for clinical trial design by enriching for subjects most likely to benefit from a therapy, and in the future, after more than one currently investigational drugs are approved, for precision medicine to identify the most efficacious therapy for high-risk subgroups.
Source: National Center for Advancing Translational Sciences
Term: July 15, 2020 – June 30, 2022
Amount: $748,638 FY